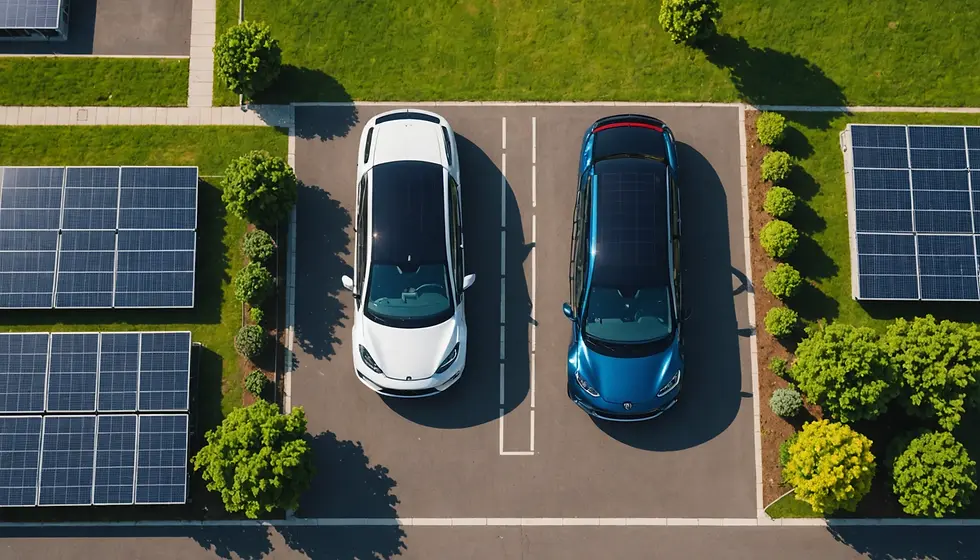
In recent years, electric vehicles (EVs) have become a focal point in the conversation around sustainable transportation. Growing environmental concerns and rapid technological advancements are driving the demand for innovative solutions that enable vehicles to be more efficient and self-sustaining. Self-charging technologies play a crucial role in maximizing the efficiency and sustainability of EVs. In this post, we will explore various self-charging technologies currently being integrated into electric vehicles.
Understanding Self-Charging Technologies
Self-charging technologies allow electric vehicles to generate their own electricity while they are in motion or parked, reducing their reliance on conventional charging methods. These technologies operate through several mechanisms, including regenerative braking, solar panels, and sophisticated battery systems.
Regenerative braking is a notable feature that recovers energy typically lost during braking. This recovered energy is redirected to recharge the vehicle's battery. For instance, studies show that regenerative braking can capture up to 70% of the energy normally wasted in braking, significantly extending driving ranges by adding extra miles for every charge.
Solar panels represent another innovative approach. By capturing sunlight, these panels can provide electricity for auxiliary functions or even charge the main battery. A practical example comes from manufacturers like Hyundai, which have integrated solar roof panels into their models. During sunny conditions, these solar panels can generate up to 600 watts, contributing considerably to the vehicle’s energy needs.
The Role of Battery Management Systems
An efficient battery management system (BMS) is essential for optimizing the capabilities of self-charging technologies. The BMS oversees the charging and discharging processes, ensuring energy is used effectively. It protects the battery from damage, and enhances its lifespan and performance.
A well-functioning BMS allows for the better integration of various energy sources. For example, if solar energy is abundant, the solar panels can be prioritized to charge the battery, easing the demand on the regenerative braking system. This strategy leads to a smoother and more sustainable energy cycle, pushing the goal of self-sufficiency in EVs closer to reality.
Emerging Technologies: A Glimpse into the Future
As the era of self-charging vehicles progresses, exciting new technologies continue to emerge. One groundbreaking development is wireless charging, which allows vehicles to charge while parked or even on the move. This technology uses induction coils embedded in the roadway to transfer energy wirelessly. Studies suggest that implementing widespread wireless charging could reduce the need for traditional charging stations by as much as 50%.
Another promising advancement involves piezoelectric materials. These materials generate electricity from mechanical stress. When integrated into roadways or vehicle components, they can turn the movement of vehicles into usable electrical energy. A pilot project in Italy demonstrated that piezoelectric systems could produce enough energy to power streetlights from the vibrations of moving cars.
Challenges and Limitations
Despite the promise of self-charging technologies, challenges remain. For example, the energy captured through regenerative braking may not be enough for long drives without the support of traditional charging. Reports indicate that on average, regenerative braking alone can provide only 10-20% of the energy needed for a full charge in many situations.
The efficiency of solar panels also varies greatly depending on weather conditions and geographical location. Some regions may see less energy generation during certain seasons, which poses a challenge for consistently self-sustaining vehicles.
Innovative systems like wireless charging and piezoelectric materials require substantial investments in infrastructure as well as technological development. A concerted effort among manufacturers, governments, and consumers is vital to overcoming these hurdles.
A Holistic Approach to Efficiency
Enhancing the self-charging capability of electric vehicles goes beyond technology; it involves a holistic view that incorporates user behavior and vehicle design. Encouraging drivers to adopt efficient driving patterns—like soft acceleration and gentle braking—can maximize the benefits of regenerative braking.
Additionally, optimizing vehicle weight is vital. By using lightweight materials, manufacturers can minimize energy consumption during acceleration and braking. Recent advancements in composite materials are paving the way for stronger yet lighter vehicle designs, ultimately improving energy efficiency.
Real-World Applications and Success Stories
Several automotive manufacturers are actively integrating self-charging technologies into their vehicles. Tesla is a notable example, having effectively utilized regenerative braking across its vehicle lineup to enhance range and efficiency. Reports indicate that Tesla vehicles can reclaim energy equivalent to approximately 15% of their total driving energy through regenerative braking.
Hyundai’s exploration of solar roof panels is another promising initiative, aiming to add supplementary charging capabilities to their EVs. These advances demonstrate that investing in self-charging technologies can capture consumer interest and drive market growth.
The Road Ahead
The future of electric vehicles is closely connected to advancements in self-charging technologies. By integrating and optimizing these systems, we can make EVs more self-sufficient and efficient. As manufacturers continue to innovate and consumers become more aware of these advancements, the potential for self-sustaining electric vehicles will continue to expand.
With new technologies on the horizon and ongoing global efforts to promote eco-friendly transportation, the future looks bright for electric vehicles. If you have any questions or need further details, feel free to ask!
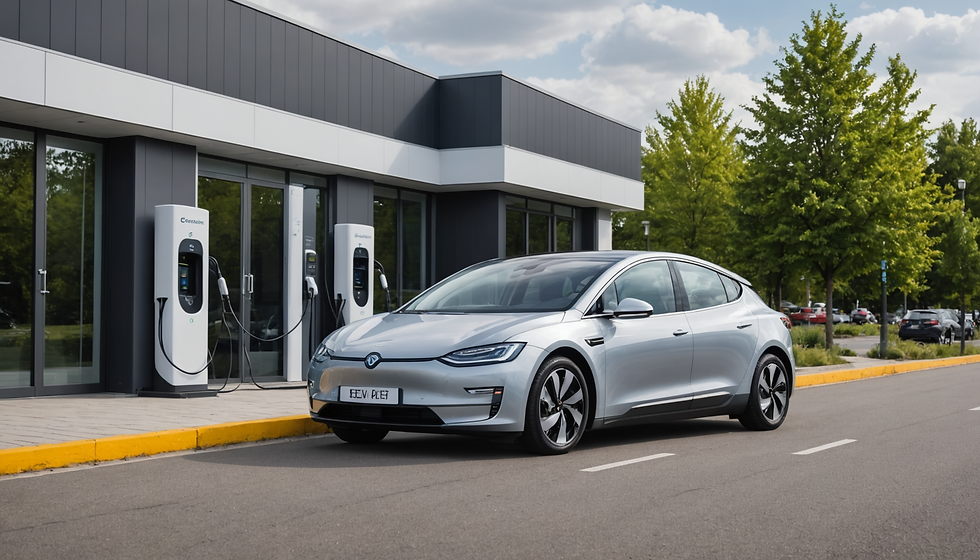
Here's an table that includes self-charging technologies for electric vehicles (EVs):
Technology | Description | Expected Output (kWh) |
Regenerative Braking | Captures kinetic energy during braking | 10-20 kWh |
Solar Panels | Converts sunlight into electricity | 1-5 kWh |
Kinetic Energy Recovery Systems (KERS) | Recovers energy from vehicle motion, especially during deceleration | 5-15 kWh |
Thermoelectric Generators | Converts waste heat from vehicle components into electrical energy | 1-3 kWh |
Piezoelectric Generators | Generates electricity from mechanical stress and vibrations | 0.1-1 kWh |
Wind Turbines | Generates electricity from airflow while driving | 1-3 kWh |
Hydrogen Fuel Cells | Produces electricity through a chemical reaction between hydrogen and oxygen | 50-100 kWh |
Biofuel Generators | Converts biofuels into electricity onboard the vehicle | 10-30 kWh |
Mechanical Flywheels | Stores kinetic energy and converts it back into electrical energy | 5-20 kWh |
Supercapacitors | Stores and releases large amounts of electrical energy quickly | 10-50 kWh |
Hydraulic Suspension Energy Harvesting | Converts kinetic energy from suspension movements into electrical energy | 0.1-0.4 kWh |
Microbial Fuel Cells | Uses bacteria to generate electricity from organic matter | 0.1-1 kWh |
Vibration Energy Harvesters | Captures energy from vibrations and converts it into electrical power | 0.1-0.5 kWh |
Radio Frequency (RF) Energy Harvesting | Captures ambient radio waves and converts them into electrical energy | 0.01-0.1 kWh |
Photovoltaic Paint | Paint embedded with photovoltaic cells converts sunlight into electricity | 1-3 kWh |
Inductive Charging | Transfers energy wirelessly from a charging pad to the vehicle | 3-11 kWh |
Dynamic Wireless Charging | Charges the vehicle wirelessly while it is in motion over specially equipped roads | 10-20 kWh |
Hybrid Energy Storage Systems | Combines batteries and supercapacitors for optimal energy storage and release | Varies |
Thermal Energy Storage | Stores thermal energy and converts it into electrical energy | 1-5 kWh |
Compressed Air Energy Storage | Uses compressed air to store and release energy | 5-15 kWh |
Electrochemical Capacitors | Stores energy through electrochemical reactions | 10-50 kWh |
Graphene-Based Batteries | Utilizes graphene to enhance battery performance and energy density | Varies |
Solid Oxide Fuel Cells | Generates electricity through the electrochemical oxidation of a fuel | 10-100 kWh |
Flow Batteries | Uses liquid electrolytes to store and release energy | 10-50 kWh |
Hybrid Solar-Wind Systems | Combines solar panels and wind turbines for continuous energy generation | 5-10 kWh |
Magnetic Induction | Generates electricity through magnetic fields | 1-5 kWh |
Thermophotovoltaic Cells | Converts thermal radiation into electrical energy | 1-3 kWh |
Hydraulic Energy Recovery Systems | Uses hydraulic systems to capture and store energy | 5-15 kWh |
Organic Photovoltaics | Uses organic materials to convert sunlight into electricity | 1-3 kWh |
Perovskite Solar Cells | Uses perovskite materials for high-efficiency solar energy conversion | 1-5 kWh |
Triboelectric Nanogenerators | Generates electricity from mechanical motion and friction | 0.1-1 kWh |
Electrostatic Generators | Converts mechanical energy into electrical energy through electrostatic induction | 0.1-1 kWh |
Biohybrid Solar Cells | Combines biological and synthetic materials for solar energy conversion | 1-3 kWh |
Quantum Dot Solar Cells | Uses quantum dots to enhance solar energy conversion efficiency | 1-5 kWh |
Thermionic Generators | Converts heat directly into electricity using thermionic emission | 1-3 kWh |
Piezoelectric Roads | Embeds piezoelectric materials in roads to generate electricity from vehicle pressure | 0.1-1 kWh per vehicle |
Nanogenerators | Uses nanotechnology to convert mechanical energy into electrical energy | 0.1-1 kWh |
Bioelectrochemical Systems | Uses biological processes to generate electricity | 0.1-1 kWh |
Thermoelectric Paint | Paint embedded with thermoelectric materials converts heat into electricity | 0.1-1 kWh |
Electrochemical Hydrogen Storage | Stores hydrogen electrochemically for later use in fuel cells | Varies |
Solar Thermal Collectors | Uses solar energy to heat a fluid, which is then converted into electricity | 1-5 kWh |
Thermophotonic Devices | Converts thermal energy into electrical energy using photonic processes | 1-3 kWh |
Electrochemical Flow Cells | Uses liquid electrolytes to store and release energy | 10-50 kWh |
Hydrogen Storage Tanks | Stores hydrogen for use in fuel cells | Varies |
Solar Windows | Windows embedded with photovoltaic cells convert sunlight into electricity | 0.1-1 kWh |
By integrating and optimizing these technologies, we can significantly enhance the self-charging capabilities of EVs, making them more efficient and sustainable. If you have any more questions or need further details, feel free to ask!
Comentários